Nuclear Power Reactor Technology, 1950-1953 (Part III)
Note: In the two previous installments (Part I and Part II), we took a look at a fascinating set of reports covering the perceived advance of nuclear power reactor technology in the earliest days of the "First Nuclear Era." This third installment completes the series, and discusses the final group study for an early nuclear power plant.
Bechtel Corporation and Pacific Gas & Electric Co.
The names above are recognizable as two that were prominent from the very earliest days (later than these studies, of course) of the actual first round of construction of nuclear plants in the United States. Pacific Gas & Electric (PG&E) owned the grid to which the early Vallecitos boiling water reactor was connected. It was a pioneering endeavor built as part of a joint effort by General Electric, PG&E, and Bechtel Corporation. Bechtel built the groundbreaking Experimental Breeder Reactor 1 (EBR-1) years before this joint effort, and eventually became the architect-engineer building the first large privately funded nuclear plant in the United States (Dresden Nuclear Generating Station).
At roughly the same time period as Dresden, PG&E commissioned its own nuclear plant, Humboldt Bay Unit 3 (a small GE BWR offered as part of GE's "Project Sunrise" program) in a project that also involved Bechtel Corporation. This study may be the spark of both companies' apparent later serious interest in commercial nuclear energy.
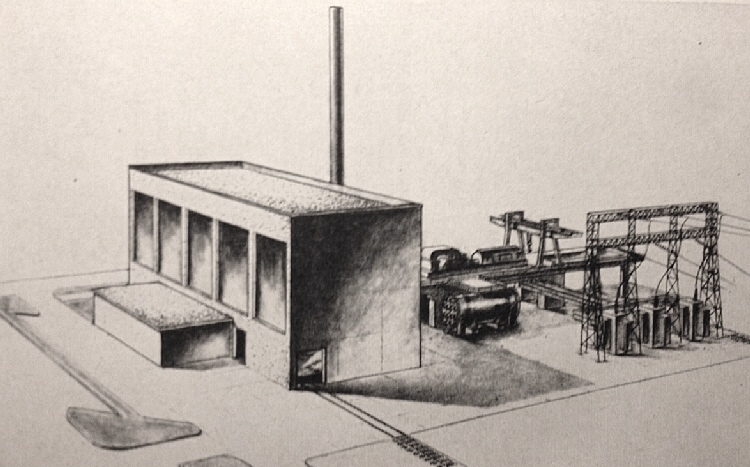
Bechtel Corporation / PG&E Nuclear Power Plant Concept, circa 1953.
Above is an illustration of a generic nuclear plant concept by Bechtel and PG&E. The reactor building is the large, rectangular structure dominating the power plant's outline. The reactor is below grade in either the water-cooled or sodium-cooled design options. To the right of the drawing is an outdoor-type of turbine generator with associated mobile gantry crane, and adjacent to that is the compact electric switchyard and transmission line tower. A railroad spur and an office structure complete the design.
The report by the two companies tells us that even at this early date, the rising demand for power on PG&E's system when coupled with the decreasing opportunities to build new hydroelectric power plants made atomic energy an attractive idea. (Of course, in this early study as with those in Part I and Part II, the power plants would all be "dual purpose"-producing plutonium for the U.S. Atomic Energy Commission as well as commercial electric power.) In a clear view toward the future, the joint study said that "atomic power, having high-capital cost and low-fuel cost, would be economically justified where it could operate at a high-capacity factor and earn maximum power revenue. Such power could well be integrated into a large system like that of PG&E, which has a large and rapidly increasing power demand, approximately one-third of which is baseload power."
The participants mentioned the clear workability of a number of contemporary government projects. Named were the EBR-1, the U.S. Navy's STR and SIR (and the project-only CVR, which would later become the Shippingport Atomic Power Station), and of course the production reactors at Hanford and Savannah River. Bechtel/PG&E felt that the proper size for such an introductory dual purpose reactor would be 500 MWt/100 to 150 MWe. They performed initial cost and feasibility studies on a fast breeder whose design was underway at Knolls Atomic Power Laboratory, an aqueous homogeneous reactor proposed by Oak Ridge National Laboratory, and a heavy water-cooled and moderated concept proposed by Argonne National Laboratory. The aqueous homogeneous design was immediately identified as not anywhere near ready, or even technically feasible in the short term, while the other two designs had shortcomings of their own. Just by this initial outlook, the prospects were bleak.
In order to complete the study for the AEC, Bechtel/PG&E selected two different designs: First, a heavy water-moderated and light water-cooled reactor that was to be made available soon (and which was much closer to the Navy's ideas than the initial first three types considered), and second, a liquid metal-cooled fast reactor not immediately feasible, but highly desirable because of its breeding potential-as well as its high temperatures and thus high thermal efficiency. The general design of power plant shown earlier (the external view) generally covers both types.
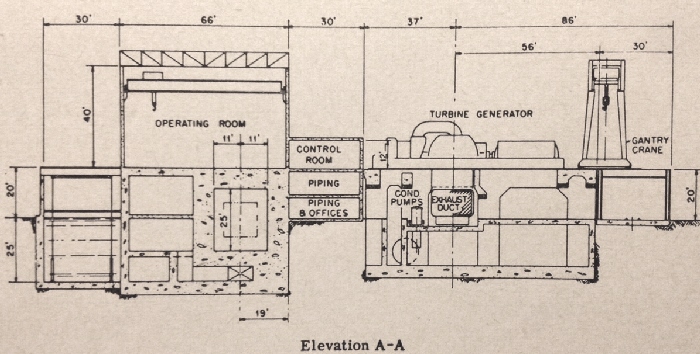
Bechtel / PG&E Nuclear Plant design, 1953.
Above, an elevation view of the general design for a nuclear plant by Bechtel and PG&E. On the left, the "operating room" is what today would be called the "refueling floor," and is above the cavity for the reactor itself (seen generically as an outline inside the right most cavity of the concrete structure). The control room for the plant is at grade, above the pipe tunnel (carrying steam to the turbine generator and returning condensate back to the nuclear steam supply system) with the outdoor type turbine generator and the gantry crane used to install and service it completing the view.
The first design was what would later become well-known as a "pressure tube" reactor. To wit: "Essentially the reactor consists of an aluminum tank, with a multiplicity of aluminum tubes between tube sheets, serving as the container of the moderating heavy water. The fuel is placed in pressure tubes which extend through the core tank concentric to the aluminum tubes. Light water, the primary coolant, flows through these pressure tubes." Four steam generators operating at 250 psig on the secondary side (and 1100 psig on the primary side) were to supply saturated steam to a tandem-compound double-flow 1800 RPM outdoor type (sealed against weather) turbine generator rated at 125,000 kwe gross. This turbine was to have outdoor, twin side-mounted condensers. Plant service power was provided by a 2300 volt bus powered either by the plant's own turbine generator or from offsite power. An emergency 125-volt battery and an engine-driven generator were to be on hand as well, and the design specified "all important instruments, plus the emergency reactor cooling pump and emergency lighting, are automatically switched to the emergency power in the event of main power failure." Indeed, in a number of ways, the design features of actual, early nuclear plants was already being predicted and set, with the exception of below-grade siting for the reactor (which, after Shippingport and Humboldt Bay used it in the United States, was not continued here.)
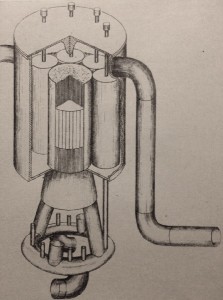
Sodium-Cooled Fast Breeder Reactor Assembly, Bechtel / PG&E study, 1953. Core at center is surrounded by rotating "control drums" which alter reflectivity to neutrons and overall core reactivity. Note ring type sodium distribution header at bottom. Plant was to use electromagnetic sodium pumps.
The second type of reactor, shown here would have been a sodium-cooled fast breeder reactor said to "produce more fuel than it uses," which made it highly desirable from a production and fuel cost standpoint. The sodium coolant was to exit the reactor core at a temperature of 900 °F, and was to be passed through two intermediate heat exchangers after which its temperature on returning to the core was more on the order of 600 °F. The second loop of sodium (to which the primary had given its heat in the intermediate heat exchangers) was then used to boil water at 500 psig and 750 °F in two steam generators. The total steam flow was to be 1,600,000 lbs/hr of steam, delivered to the throttle of the turbine generator at 485 psig. The turbine generator used on this design was to be rated 154,600 kwe and was also an 1800 RPM unit.
The participants knew the varied technical problems that sodium-cooled reactors faced, and were plainly honest about the hurdles, estimating that at least two further years would be required to complete a power plant of this type as compared with the water-cooled reactor. Further, "...it is important to recognize that the liquid metal-cooled reactor cannot be considered equal to the water-cooled thermal reactor on the basis of applicable existing technology, time required for design and construction, reliability of cost and performance estimates, or safeguards and degree of isolation now required." This all holds true today over a half century later; the two companies had properly augured the future in favor of the light water reactor as a commercial power machine, probably without knowing it.
Postscript
None of the reactors we've seen in this three-part series was built. The guess on the part of participants that very high temperature reactors would eventually be more economically viable than water-cooled reactors, because of higher efficiency, was fundamental to the early concepts of nuclear energy and not out of line with the revelations even at this early date that water-cooled reactors were "available now or almost immediately." No commercial, civilian-owned reactors were ever built in the United States that adopted the dual power production and plutonium production concept, either, as immediately the commercial nuclear era would be entered quite another way. The briefly mentioned CVR project was cancelled by the U.S. Navy, adopted wholly by the AEC and turned into the Shippingport Atomic Power Station. This event and others, such as the CAP (Civilian Application Program, see here and see here and finally see here), ushered in a true private ownership and operation of nuclear plants apart from AEC ownership (eventually) and skirted any need to have the dual cycle nature embodied in all the plants we've looked at here. Nevertheless, the designs are fascinating to consider in hindsight, not only for their completeness at such an early date but also for the projections they made on the future.
Reference: "Reports to the US Atomic Energy Commission on Nuclear Power Reactor Technology." US Government Printing Office, Washington, D.C. May 1953.
Will Davis is Communications Director and board member for the N/S Savannah Association, Inc. He is a consultant to the Global America Business Institute, a contributing author for Fuel Cycle Week, and he writes his own popular blog Atomic Power Review. Davis is also a consultant and writer for the American Nuclear Society, and serves on the ANS Communications Committee and will serve on the Book Publishing Committee beginning in June. He is a former US Navy reactor operator, qualified on S8G and S5W plants.